Ça y est… the CEReS project has come to an end. A summary of the current project is below, while we continue to consider the next steps…
Executive Summary
Many coal mines produce waste which causes acid mine drainage (AMD) potentially resulting in severe environmental damage. This drainage can be treated, but most wastes will continue to produce such drainage for hundreds of years. At the same time, the pace of technological development means most electrical and electronic equipment becomes obsolete within a matter of years, resulting in the generation of vast quantities of electronic waste (e-waste). Where this cannot be recycled, it must be discarded. The CEReS project sought to develop a co-processing approach to treat these waste streams to produce metals and other valuable products, while eliminating their environmental impact. This brings together two waste streams from opposite ends of the supply chain; turning each into a novel resource in a single, coherent ‘grave-to-cradle’ process. This industrial ecology approach is key to supporting a circular economy while securing the sustainable supply of critical raw materials. The project successfully elaborated a novel co-processing flow-sheet comprising: (i) the accelerated weathering of AMD-generating coal production wastes to generate a biolixiviant; (ii) the pyrolysis and catalytic cracking of low-grade PCBs to produce hydrocarbon fuel, a halogen brine a Cu-rich char; (iii) the leaching of base metals from the char using the biolixiviant; (iv) the reuse of the stabilised coal wastes; and (v) the recovery of valuable metal while concentrating precious and critical metals into enriched substrates.
Introduction
The CEReS project received funding from the Research Fund for Coal and Steel (RFCS), a funding body administered by the European Commission, but outside of the Framework Programme. The fund provides 50 M€ every year to “innovative projects to enhance the safety, efficiency and competitive edge of the EU coal and steel industries”. The total budget was approximately 3.2M€, and the project comprised eight partners from five countries. Poland was chosen as a case study region, due in part to its substantial coal mining activity and challenges with subsequent wastes and relatively underdeveloped e-waste recycling sector. The project set out to demonstrate the technical feasibility of the individual unit processes at laboratory/pilot scale. These were then integrated in silico, and an economic assessment made. An LCA approach was used to evaluate the environmental benefits of the CEReS process compared to the business as usual, do-nothing scenario.
Poland is Europe’s largest producer of hard coal, producing ~66 Mt of coal pa; coal is used in the generation of approximately 80% of the country’s electricity. As a result of this mining activity ~25 Mt coal production waste is generated pa, much of which contains varying levels of pyrite. While 90% is reused in civil and geotechnical engineering, there are problems where this material is subject to weathering and the dissolution of the pyrite. Beyond this, there are significant stockpiles of historic mine waste. Tauron Wydobycie S.A. (Poland) own the Brzeszcze, Janina & Sobieski coal mines and hold the largest coal reserves in Poland. These mines produce sulfidic wastes, much of which are currently stock-piled. The company is actively investigating alternate routes for wastes to improve economic and environmental performance; this necessitates a long-term solution to AMD-generating wastes.
EU reliance on importation of critical raw materials (CRM) is well-documented. At the same time, the importation of electronic and electrical equipment (EEE) is an “open-loop”; a net influx of CRM to non/minimally-producing regions. Therefore, waste EEE (WEEE) represent an important reserve of CRM. However, the recycling of such end-of-life consumer products is complex, requiring sophisticated disassembly, separation and recovery processes.
Comet Traitements (Belgium) cover the entire recycling and valorisation chain. Currently they recover 97.8 % of end of life vehicles for reuse. As part of this process, they developed a pyrolytic process to treat the organic fraction of light shredder residues (LSR) producing hydrocarbon fuel, carbon-rich concentrates and metal-bearing char. Comet wish to broaden the application of this process to scrap printed circuit boards (PCBs) from electronic waste.
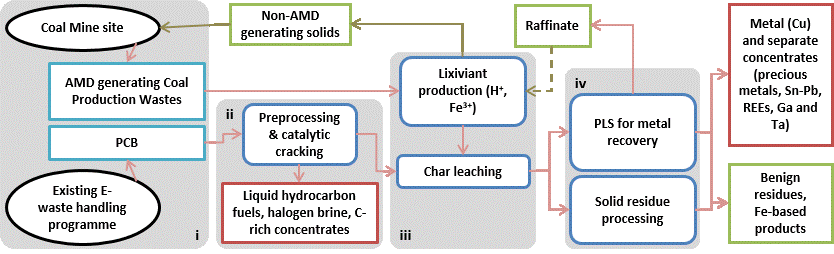
Figure 0.1: Simplified overview of the proposed CEReS co-processing flow-sheet.
The proposed CEReS process is an example of industrial ecology. It brings together two waste streams from opposite ends of the supply chain, turning each into a novel resource in a single, coherent ‘grave-to-cradle’ process. The proposed co-processing flow-sheet is summarised in Figure 0.1, and can be considered in four parts:
- Raw Materials. AMD-generating coal mine wastes are recovered from existing mine waste dumps or during ongoing production. PCBs (shredded) are bled off from existing waste electronic and electrical equipment (WEEE) or e-waste handling streams. Therefore, there are no mining or comminution costs associated with the supply of raw materials for the CEReS process.
- Pyrolytic Cracking. A catalytic cracking circuit is used for pre-treatment of the PCBs resulting in a metal-rich char. Catalytic cracking partially converts the organic fraction into a liquid fuel (which can be used for electricity generation for downstream processes) and a carbon-rich solid residue (with potential applications as a reducing agent in metallurgy or steelmaking). A quenching process removes halogens (mostly bromine) as a saleable brine. The low temperatures and reducing conditions prevent the formation of dioxins while maximising product recovery.
- Lixiviant Production and Char Leaching. A bioreactor system is used to oxidise sulfide minerals in the coal wastes (effectively accelerating AMD production), resulting in the production of a ferric iron-sulfuric acid lixiviant. This is used to leach base and other soluble metals from the PCB char. The stabilised coal wastes (depleted of their AMD-generating potential) can be used, for example, as backfill in rehabilitation of the mine site or in civil engineering (aggregates).
- Metals recovery and process residues. Valuable metals (Cu and potentially REEs, Ga and Ta) are recovered from the pregnant leach solution (PLS) by appropriate downstream process and the raffinate recycled to the coal waste bioleaching reactor (to maximise efficient process water use). This circuit will include a system for managing the iron content, as significant amounts will be encountered throughout the process as a whole. Precious metals, lead and tin will report to, or remain in, the solid leach residue and will be physically separated to produce concentrates suitable for established refining metallurgy.
Raw Materials
Coal production waste
Four waste types where considered from the two mines, Janina and Sobieski: sludge (-0.1 mm), spiral tails (0.1-2 mm), jig tails (2-20 mm) and heavy liquid tails (20-200 mm). The sludge and heavy liquid tails were discounted due to their low sulfide content and difficulty with handling (either too fine or two coarse). The jig and spiral tails from Janina (JJT and JST, respectively) and Sobieski (SJT and SST, respectively) were extensively characterised. Detailed mineralogical analysis necessitated the development of a novel QEM-SCAN protocol. Finally, the Janina spiral tails were selected for the project due to their high pyrite content (approx. 12%). However, subsequent QEM-SCAN analysis indicated limited liberation of the pyrite (25-30%). Chemical and biokinetic tests confirmed that the selected material was acid-generating. However, far less acid was produced given the amount of sulfide oxidised (in both AMD tests and bioleaching experiments); this “missing acid” has yet to be explained.
Currently, the Janina coal production waste is stockpiled on site. Samples were collected from the waste heap at the Janina mine in late 2016. These were used for microbial enrichments. Attempts were made to extract DNA from these samples with little success, indicative of relatively low microbial cell numbers. However a more comprehensive sampling campaign was undertaken comparing geochemical observations with high throughput DNA (16S rRNA gene) analysis. This analysis revealed a community dominated by strains typical of metal rich, low pH environments (e.g. Acidithiobacillus spp., Leptospirillum spp., Sulfobacillus spp., etc..), but a large fraction typical of saline environments (e.g. Acidihalobacter spp.).
Scrap printed circuit boards
The Polish WEEE market was analysed, and three categories of scrap PCBs were identified: high-, medium- and low-grade. The low-grade material was selected, but analysis found it was in fact a mixture of PCBs (~35%) and other parts of WEEE. Therefore, for the development of the project, Comet used low-grade PCBs from their own stocks. This material was analogous to the scrap PCBs available on the Polish market. Detailed analysis showed that copper represented approximately 23% of the intrinsic PCB value while precious metals represented over 60%. The CEReS project work focussed primarily on the recovery of copper, but further work should be under-taken to recover precious metals.
Notwithstanding this classification, Umicore would still buy these PCBs; they are not below their minimum purchase valuation. For CEReS this means that there exists a processing option for these low-grade PCBs, putting CEReS in competition with existing processes. This was not originally foreseen and must be considered in the evaluation of the CEReS business model.
Cross-mapping
A database was created cross-mapping the occurrence of suitable mine wastes (dumps and mining operations) with WEEE handling facilities. This allowed the identification of geographically-suitable potential locations for a CEReS process. The database also contains data on sulphur contents in mining waste produced in coal processing plants and enabled the visualisation of information on the potential processing of electronic waste in particular waste management plants.
Three zones of distances were determined for an example coal production waste heap, which is located near the Janina mine in Libiąż. In a zone within 150 km from a potential source of coal production waste there are 35 e-waste processingplants (60.3% of all the plants); within 150 – 250 km from a source of coal production waste there are 15 plants (25.9% of all the plants); and within 250 – 350 km from a source of coal production waste there are 13 plants (13.8% of all the plants). From an operational point of view, there is a good degree of existing co-location of coal mine and concentrations of electronic waste in the target region.
Pyrolytic Cracking
Bench- and pilot-scale work at Comet successfully adapted the pyrolytic process to shredded scrap PCBs. Approximately 18% of the PCB mass could be converted to liquid and gaseous combustible hydrocarbons, the calorific value of which is sufficient to power the pyrolysis reactor at industrial scale, making the process energy-neutral. Labs tests found that approximately 75% of bromine content of PCB is released from the solid residue of the pyrolysis into the quench water, from which it is possible to produce a saleable Br product. A recovery method to extract bromine from the liquid hydrocarbon was tested, resulting in removing 69% of its bromine content. The metal-bearing char from the process was split into four size fractions: coarse (>8 mm, 6.2% Cu), mid (2-8 mm; 56 % Cu) fine (75 µm-2mm; 35% Cu) and very fine (<0.075 mm; 4.2% Cu). The mid fraction was sufficiently Cu, Au and Ag-rich that it can be sold directly to existing metal refiners. The very fine fraction can be disposed of conventionally while the remaining fractions are the target for char leaching using the biolixiviant.
Lixiviant Production and Char Leaching
Bioleaching and lixiviant production
Enrichment cultures were grown at 30, 42 and 48°C from samples from the Janina waste heap. Interestingly, an active bioleaching culture could not be obtained from the 42°C enrichment, theorised to be linked to the observation of low levels of Leptospirillum ferriphilum in the waste material. Two enrichment cultures at 30°C (TW30) and 48°C (TW48) were compared to two consortia of proven bioleaching ability: meso (30°C) and BRGM-KCC (42°C). Following extensive tests, the TW48 culture at 48°C was selected. Interestingly, there was a progressive loss of Leptospirillum spp. from 42°C tests (BRGM-KCC) during scale-up. The reasons for this could not be clearly explained, but may be linked to the dissolution of steel components in the bioreactors, discussed below.
A suitable biolixiviant could be produced from the coal production waste, containing approximately 10 g/L Fe3+. However, there was significant damage to the metallic components of the bioreactors at lab and pilot scale. It is presumed that this was caused by the chloride content of the waste. It is possible to wash the waste to remove the chloride, but this would inevitably affect the process economics. On the other hand, lab-scale tests have shown that the Sobieski material is also amenable to bioleaching without the chloride issues.
Char leaching
Two reactor designs were tested: a typical stirred-tank reactor (STR) and a rotating drum reactor (RDR). The RDR comprises a horizontal mesh drum containing the char, which rotates within a sealed outer drum. Various operating conditions and char pre-treatment options were tested. Finally, an attrition grinding pre-treatment followed by wet separation was used to prepare the char for leaching. The best performance was obtained with an STR set-up, but this was not suitable for the larger (2-8 mm) char fraction. Conversely, the RDR was not suitable for leaching the finer fractions as the char was not retained within the inner mesh drum. Therefore, the RDR was selected to leach the coarse fraction and an STR for the fine fraction.
The dissolution was bi-phasic, with a rapid initial dissolution of up to 40% of the copper over a few hours followed by a much slower dissolution phase. One possible explanation could be the formation of oxidised copper phases after the pyrolytic step (during quenching or attrition grinding, for example). However, it is more likely linked to the stoichiometric ration of ferric iron to oxidisable metals. While there was some evidence of microbial colonisation of the char leaching reactor, the benefit of this to the process was not conclusively proven. Maintaining microbial activity (iron-oxidation) in the char leaching reactor could help overcome the stoichiometric limitations and lead to more efficient char leaching. Nevertheless, 100% of the copper could be leached from the char using the biolixiviant solution.
Metals recovery and process residues
Metal recovery
The leachate from the char leaching step is a complex mixture of metals. Therefore, the highly selective Acorga reagent was chosen for solvent extraction (SX). A two-stage mixer-settler configuration was proposed followed by electrowinning (EW) which could produce copper cathodes. Direct electrowinning was considered, but discounted given the high iron content of the leachate. Iron management was possible via the precipitation of jarosite and autoclave conversion to hematite.
Within the scope of the project, it was only possible to valorise the copper, with the precious metals sold to existing process within the leached char residue. However, future work will look at valorising this important fraction within the CEReS process.
Process residues
Environmental toxicity tests indicated that the leached char was less of a threat to the environment than the PCBs prior to treatment. The sulfide component of the coal production waste was almost completely removed following bioleaching. Accordingly, the maximum potential acidity (MPA) was reduced. While the amount of sulfate produced during net acid generation tests (NAG) suggested the material was non-acid-forming, the pH of the solution became acidic. Furthermore, negative acid neutralising capacities (ANC) were observed. Taken together this suggests that the material may be leaching acid, but not through the oxidation of residual sulfides. It may be that the ‘missing’ acid is adsorbed to the material and may desorb in the environment.
Four options were considered for the reuse of the leached coal waste: ceramic products (bricks), production of granulates, and concrete and polymer-concrete products. The addition of leached material (15% initial mass) during brick production had an effect on the final colour, but produced satisfactory bricks with slightly elevated compressive strength. Incorporation into cement products (15%) again had no detrimental effects and again slightly improved compressive strength. Concrete polymer products could be made from the waste alone. However, this required a long bonding time rendering this option for reuse impractical.
Finally, the leached waste was tested as a substrate for the production of granulates. The granulates produced were of high strength and tests confirmed that leachate produced did not exceed permissible contaminant levels. Therefore, such granulates can be safely used in underground mining techniques, civil engineering and could even be used for combustion (as the waste contains sufficient residual coal).
Economic and Environmental Assessment
Process integration through simulation
A global simulator created using USIM PAC based on the overall process flowsheet, allowed an economic assessment and evaluation of alternative flow-sheet options. Based on the pyrolysis of 14,000 t/y of PCB, CEReS would require (and reprocess) 79,000 t/y Janina coal waste producing 76,000 t/y stabilised waste for reuse, 2,100 t/y enriched char for direct sale to existing processing operations, 923 t/y coper cathode and a final residue containing the precious metals.
The total power demand was estimated to be around 3.9 MW. The main energy-intensive step is the heating of the pregnant leaching solution to 90°C for iron precipitation. Therefore, this option was replaced with simple precipitation using lime. The second energy expenditure item is the first bioleaching tank, mainly due to thermal regulation because of the highly exothermic behaviour of the pyritic coal waste bioleaching system. The energy required for coal waste milling in a ball mill is significantly lower than the energy required for downstream bio hydrometallurgical operations.
Based on a PCB market value of 1,600€/t, reprocessing coal mine waste using CEReS would cost approximately 93 €/t. Such a value is in line with European costs for landfill disposal of this type of waste, but is much higher than the current costs in Poland. However, the current simulation assumes that the stabilised wastes have no value, which may not be the case if, for example, the ceramic or concrete products could be sold. Furthermore, the majority of the PCB value is in the precious metals and the current flowsheet does not include a model for their valorisation. This will be further developed in the future. Overall, there is a need to improve process efficiency and secure an income for mine waste reprocessing, e.g. negative cost for mine waste.
Life cycle analysis (LCA)
A comprehensive LCA of the proposed flowsheet revealed that CEReS was more environmentally friendly than the current “do nothing” scenario in almost all categories. The exception was categories linked to fossil fuel use. This is due to the fact the CEReS is a relatively energy-intense process and Poland’s (the case study country) energy mix is carbon-heavy. Nevertheless, the environmental benefits of CEReS are clear.
Conclusion
The project successfully demonstrated the key parts of the CEReS flowsheet at lab/pilot scale. The process was able to convert scrap PCBs into combustible fuels, a halogen brine and a metal-rich char. AMD-generating coal production waste could be stabilised through accelerated wreathing and reused in construction products. The lixiviant produced was suitable for leaching the copper from the char, and this could be refined into copper cathodes. In silico integration and simulation demonstrated the technical viability of the process. The economic assessment, at present, is unfavourable; the process does not create sufficient value to cover the operating costs. While this may improve with improved processing efficiency, and certainly if steps are included to recover the precious metal content, profitability is not necessarily at the heart of the CEReS business model. The LCA approach validated the environmental advantages of reprocessing such wastes in this way over the current do nothing scenario. The extractive industry, and society at large, is facing increasing pressure to find and develop long term, sustainable solutions to handling mine waste. The CEReS process permanently removes the AMD-generating potential from mine wastes, opening the possibility for their reuse, or at least environmentally benign storage. The value recovered by co-processing with scrap, low-grade PCBs off-sets the mine waste reprocessing costs. The challenge is to improve processing performance so that this cost per ton is less than the current best available options.
Recent Comments